Hearts & Bioprinting
3D Bioprinted Models to Assist Planning of Complicated Cardiac Surgeries
The heart is one of the most complex organs of the body. It is responsible for pumping blood to all the parts of the body so that our tissues can get oxygen and for receiving impure deoxygenated blood from the body and sending it to the lungs so that the oxygen can be replenished. It is also responsible for making sure that pure oxygenated blood doesn't mix with impure deoxygenated blood.
In a healthy heart, impure blood and pure blood are kept in the right and left chambers of the heart, respectively. A septum (a wall) prevents the blood in the two chambers from mixing. The aorta carries blood from the heart to the rest of the body, while the pulmonary artery carries impure blood from the heart to lungs for purification.
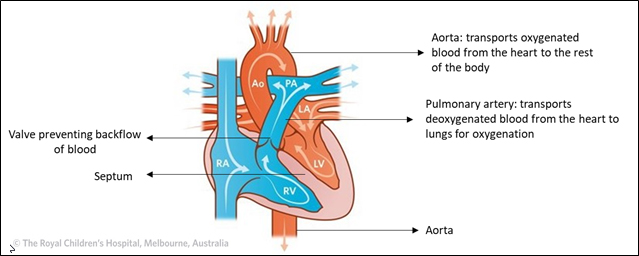
Normal Circulation
Adapted From: The Royal Children’s Hospital, Melbourne, Australia
However, some rare congenital heart defects lead to a disruption of this normal flow. Tetralogy of Fallot with major aortopulmonary collateral arteries (TOF/MACPA) is one such congenital heart defect. TOF patients have a defective septum allowing blood from the right side of the heart to freely flow into the left side and pass into the aorta and to the rest of the body, even though it has not passed through the lungs for oxygenation. Additionally, in these patients, the opening of the pulmonary artery is blocked, sealing off the path deoxygenated blood takes from the heart to the lungs. In order to get blood to the lungs, the body of TOF patients often develops a workaround, forming extra branches from the aorta – called collateral arteries – that go to the lungs. Despite the branches, the body often does not get as much oxygen as it needs. While symptoms among patients can vary, many have difficulty breathing and have blueish skin due to a lack of oxygen.
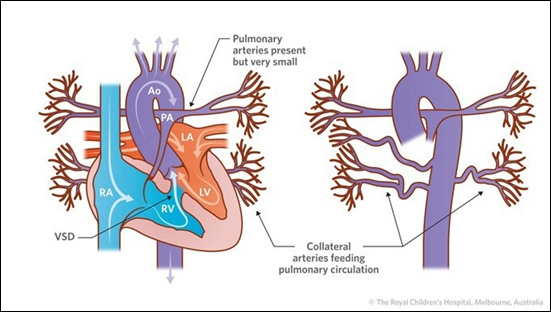
Tetralogy of Fallot with Major Aortopulmonary Collateral Arteries
Source: The Royal Children’s Hospital, Melbourne, Australia
According to Holly Bauser-Heaton, MD, PhD, an interventional pediatric cardiologist at Children's Healthcare Atlanta and an assistant professor of Pediatric Cardiology in the Emory's School of Medicine, the only treatment for TOF/MAPCA available is what is called a unifocalization surgery in which doctors try to unite the blood vessels that branch off the aorta to establish normal circulation. These surgeries are complicated and "can last anywhere between 8 to 16 hours," she says.
However, the surgery alone is rarely enough. "Part of the issue is that even if you have pristine technical skills in the operating room, these pulmonary arteries can still have the propensity to shut down or stenose over time" Bauser-Heaton explains.
Adding to the hurdles in developing better interventions is that fact that the disease is . Only 1 in 3,000 newborns are diagnosed with TOF, due to which, surgeons do not see enough patients to develop better surgical strategies or have a big enough sample size to predict which arteries will later develop constrictions.
Bauser-Heaton's collaboration with Vahid Serpooshan, PhD, an assistant professor at Georgia Tech's School of Engineering and Emory's School of Medicine, hopes to address some of these problems. An expert in 3D bioprinting, the Serpooshan/Bauser-Heaton team has developed three-dimensional models of the anatomy of individual patients based on data from MRI and CT scans that will help surgeons not only better plan the unifocalization surgery, but also potentially prevent or reduce stenosis.
Unlike most models that are 3D printed using rigid polymers like resins, Serpooshan/Bauser-Heaton's lab has been able to make these models with soft polymers, such as hydrogels. "The drawback [of current technologies] is that the mechanical properties aren't there," says Martin Tomov, PhD, a postdoctoral fellow in the Serpooshan/Bauser-Heaton Lab. "You can have a plastic heart model that is really well represented but you can't really do anything functional on it. You can practice putting catheters on it, the wires, but it would be different to what a surgeon would experience in a patient because you can't puncture a vessel that is plastic. The mechanical properties of this model are more similar when compared to patients' interstitial tissue," he explains.
In addition to ensuring that the model mimics the properties of real blood vessels, Serpooshan/Bauser-Heaton's team connects the model to bioreactors that pump a culture medium through it, simulating the way the heart pumps blood through blood vessels. Finally, cells are inserted into the model blood vessel. These cells attach to the hydrogel polymer and start growing as they would in a patient.
"Since we are working with a highly accurate anatomical model of the patient, we can study how fluids flow in the 3D printed structures and how they could interact with the cells that are now sitting and growing in the inner space of these vascular structures. It pretty much recapitulates the disease conditions that would be happening in the patient's body, except that now you can actually create hundreds of the exact same sample with high reproducibility," says Serpooshan.
These models can help surgeons try various surgical techniques without the worry of hurting a patient. According to Bauser-Heaton, surgeons typically approach unifocalization surgeries with the aim to try to reconnect blood vessels so that circulation is established but are not able to consider how the connections they make might lead to the constriction of these vessels in the months and years after the surgery.
"We have been at a stand-still for many, many years because we have been thinking about it in terms of plugging things together so that the patient survives, but I think it needs to be much more sophisticated than that and our model allows us to study that," Bauser-Heaton explains.
Changing the diameters of blood vessels or the angles they make can potentially reduce the need for future corrective surgeries. Surgeons can alter the configuration of vessels in the model, add different pharmaceutical compounds to the culture, or try other interventional measures and study how the cells respond and monitor whether the vessels are closing up. "This will allow us to do better surgical planning for sure so that we can reduce morbidity long term and repeated operations can be avoided," Bauser-Heaton says.
In addition to improving surgical outcomes, Serpooshan and Bauser-Heaton hope that these models can be used to develop therapeutics to treat TOF patients. "These are super sick babies and they have to come back to the clinic every month or so because they develop another stenosis, usually they do not survive for too long," Serpooshan says. "If we could come up with a [therapeutic] approach and can avoid formation of stenosis, that will be hugely advantageous.
"Currently, Bauser-Heaton and Serpooshan are working with Emory's Office of Technology Transfer to establish a path to develop this technology on a larger scale. "This project is an archetype of collaboration between physicians and engineers, working together to solve an unmet clinical need," commented Sat Balachander, licensing associate. "We're excited to work with the team and external partners to advance this technology to market."
Techid: 18209, 19177
Read our technology brief