Improving Vaccines
Unlocking the Secrets of Human Plasma
Anyone who's ever tried to examine a snowflake will know the challenge: the snowflake melts before you can make out the crystalline details. There is not enough time to even take a picture. It's a challenge researchers face when studying human plasma. These cells cannot survive in a petri dish for more than a few days, making them near-impossible to study.
Frances Eun-Hyung Lee, MD, an Assistant Professor of Medicine at Emory's School of Medicine, has developed a way to keep plasma cells alive in a culture dish longer than ever before. This innovation, which is seen by some as a "game changer," will open up a new range of possibilities for research, with far-reaching applications such as treating multiple myeloma and preventing fatal immune reactions from transplants.
Lee has long wondered why some vaccines seem to last longer than others. Although vaccines can provide years of protection from specific diseases, the effects often wear off over time and require supplemental booster shots. "Why aren't you protected for your whole life?" she asks.
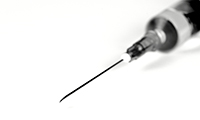
The answer may be found in long-lived plasma cells (LLPCs). Plasma cells are a type of white blood cell, a critical component of the immune system. When the body faces infection, plasma cells release antibodies, proteins which help to destroy germs. Each type of antibody is used to destroy a particular illness. Plasma cells remember the germs that they have been exposed to, via infection or vaccine, so that they can recognize and destroy the germs if they ever return. But when a plasma cell dies, the memory of the infection dies with it. Most plasma cells are short-lived, but LLPCs can survive for decades, preserving the memory of the infection and thereby protecting the individual. By studying these cells, scientists like Lee hope to develop better vaccines and treatments for immune disorders.
However, it is notoriously difficult to study LLPCs. Scientists struggle to isolate them from blood and bone marrow, and struggle to keep them alive in a lab setting. These cells were made to thrive inside the body, not inside a petri dish, and often die after a few days. This incredibly short life span greatly limits the research that can be conducted on them. Lee is "trying to recreate the bone marrow…in a culture dish," and developed a special media that can prolong cell survival to up to sixty days.
Lee began by investigating what conditions are required for LLPC survival in the bone marrow. Noticing that they are closely taken care of by mesenchymal stem cells (MSCs), which Lee describes as "nursing cells." Noticing that LLPCs could grow without direct contact with the MSCs, she postulated that LLPCs are kept alive not by the MSCs themselves, but by a substance the MSCs produce. Lee calls the substance produced by MSC the "secretome."
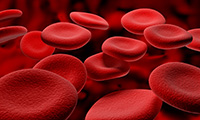
Lee tried to grow LLPCs in a petri dish mixed with the secretome, but saw only limited improvement. Around this time, she noticed that APRIL cells are also critical to LLPC survival. When she combined APRIL cells and the secretome together into the petri dish, LLPC survival skyrocketed. She was able to increase cell life span even more by reducing the concentration of oxygen in the dish, creating a hypoxic environment similar to what is found in the bone marrow where these cells naturally thrive.
This special media has multiple applications in the health field. In addition to expanding research opportunities, Lee hopes that the media will be used to create vaccines. When someone survives an infection like Ebola, immune cells remember the antibodies used to destroy the invading germ. Each person accrues countless types of antibodies over the course of a lifetime. But if doctors know that one patient survived Ebola—and no one else has—then that one person must have, hidden between thousands of irrelevant antibodies, the one type of antibody that is the secret to beating the infection. Lee hopes to sample immune cells and grow them in her media, allowing cells to produce thousands of possible antibodies, and then test those antibodies to see which one protects against Ebola. Once this antibody is identified, it can be put in a vaccine to prevent future illness. This expensive strategy of isolating antibodies usually takes about 80 days, but with Lee's media, it takes only 14 days. And in an outbreak, time is critical.
She wants to use a similar strategy to test whether a person has antibodies to a given virus, and thus requires another vaccination. "We can assess vaccine health in terms of personalized medicine," she says, seeing if that person's body remembers an illness it was vaccinated against many years ago. This technology can also help facilitate successful organ transplants. One of the most dangerous parts of a transplant is the risk of rejection, when the recipient body does not recognize the donated organ and proceeds to attack it. In order to prevent fatal immune reactions against a new "foreign" organ, doctors must carefully monitor what antibodies the body is producing against the organ, identify where they are being produced, and stop them before they reach the bloodstream. Once rejection antibodies reach the bloodstream, the immune reaction becomes increasingly difficult to control.
“Vaccines – and the protection they afford – is a pressing topic in both the scientific and lay communities. This technology combines research and clinical interests, and can provide life-saving care into the foreseeable future,” said Mark Coburn, Director, Licensing at OTT.
Lee has already allowed labs across the country to begin experimenting with her media. All of them have validated the technology as effective, and are clamoring for more. This is the "ideal technology to study the antigen repertoire that people have never really studied before," Lee adds. The possibilities are endless.
Techids: 15057, 17091
View our technology brief