Microarray Biosensors
Small Sensors Lead to Big Changes in Understanding Neuromuscular Activity
The brain is Grand Central Station when it comes to controlling the activity of every part of the body. We can do everyday activities like smiling, breathing, or drinking a glass of water without much thought, but complex mechanisms are at work to make those small actions happen.
It’s an important job that we know little about, which is why scientists – like Emory associate professor of biology Samuel Sober, PhD – are working to understand the brain-muscle connection in finer detail.
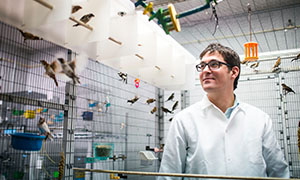
Samuel Sober, PhD
“In order for you to move your body, your brain first must send electrical signals to neurons in your spinal cord, which in turn sends electrical signals to your muscles that makes them contract,” explains Sober, who is also director of the Simons-Emory International Consortium on Motor Control. “Learning to perform new motor skills is one of the essential things that the brain does but we know next to nothing about how that process works.”
Getting the big picture
One source of inspiration for this work is Bengalese finches and their songs.
“Songbirds are the best model system for understanding how the brain controls complex vocal behavior, and one of the best systems for understanding control of motor behavior in general,” says Sober.
Sober’s lab is working at the forefront of precisely describing the computational signals, or the language, that the brain uses to control muscles. He’s particularly interested in how the brain learns and relearns motor skills — for example, in a recovering stoke patient.
Currently, clinicians use electromyography, or EMG, as a tool to diagnose the health of muscles and the motor neurons that control them. An EMG involves the use of a tiny wire, or electrode, inserted into a muscle to record the electrical activity in that muscle.
“The problem is that the readings provided by traditional EMG technology are relatively crude,” Sober says. “They provide a summary of the activity of large groups of motor neurons.”
Zooming in on the fine details
Sober wanted a more precise resolution of data for his research on animal models into how the brain activates and control muscles. His lab collaborated with the lab of Muhannad Bakir, PhD, an electrical engineer at Georgia Tech, to come up with technology to advance the neuroscience of motor activity.
The research team developed flexible, multi-electrode arrays, each containing one or more “threads” about as wide as a human hair, for recording neuromuscular activity.
“Basically, the devices are so tiny that they no longer function like a sheet of plastic, but like a suture thread that you can sew into a muscle,” Sober explains. “You can also load them into a syringe and inject them into a muscle, so the technology is minimally invasive.”
With this innovation, researchers can now record high-resolution data, at the level of a single cell, across large groups of muscles simultaneously while subjects perform complex behaviors.
“We are finally able to see data that was invisible to us before — the activity of single neurons across an entire ensemble of muscles involved in a complex movement,” Sober says. “This information is like the missing link for trying to understand how the brain controls behavior.”
The inventors have adapted the microarray biosensors in different sizes and shapes so that they can record neuromuscular activity in a variety of animal models, from songbirds and mice to monkeys and now humans. “We’re providing a platform to allow researchers to reveal the mechanisms of motor agility across the tree of life,” says Sober. “Gathering and sharing information across species is speeding up discoveries in the field of neuroscience.”
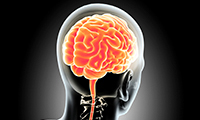
Looking toward future applications
Experiments using the microarray biosensors on animal models are already yielding new insights into the basic mechanisms of neuromuscular control. “It used to be thought that individual motor neurons conveyed only very simple signals about whether a muscle should be ‘on’ or ‘off,’” Sober says. “We’ve now shown that individual motor neurons use very precise codes in precisely timed patterns to control behavior.”
“The design for these microarray biosensors is innovative and serves an unmet need in neuroscience,” says Catherine Murari, licensing associate in Emory’s Office of Technology Transfer, explaining why OTT filed for a patent for the devices. “The first experiments involving humans implanted with the microarray biosensors are now underway, marking another major step forward.”
Comparing data from across species will help speed discoveries of the normal functioning of the neuromuscular system. That will lay the groundwork for using the high-tech biosensors as a tool in clinical settings.
Sober notes the lack of objective biomarkers to precisely measure motor control – which can determine the effectiveness of treatments for recovering stroke patients and neurodegenerative illnesses such as amyotrophic lateral sclerosis (ALS).
“The sensitivity of our microarray biosensors could potentially measure any improvement a patient may be experiencing after taking a drug or other therapy,” Sober says. “The devices may also enable doctors to diagnose a neurogenerative disease earlier so interventions can start sooner.”
Techid: 21162
Read our technology brief